- The number of electrons in an electrically-neutral atom is the same as the number of protons in the nucleus. Therefore, the number of electrons in neutral atom of Phosphorus is 15. Each electron is influenced by the electric fields produced by the positive nuclear charge and the other (Z – 1) negative electrons in the atom.
- A neutral phosphorus atom in a grounded state will have six electrons occupying orbitals in s sublevels across its electron shells.
- Bohr Model of Phosphorus Neon Atom Model, 5th Grade Science Projects, 8th copper bohr diagram wedocable - 28 images - copper element protons. As was mentioned before, a neutral Phosphorus Atom contains five valence electrons in the third shell. You can see a Bohr Model of Phosphorus.
- Electrons are locked up in covalent bond with valence electrons of four neighboring Si atoms. However, the 5th valence electron of phosphorus atom does not find a binding electron and thus remains free to float. When a voltage is applied across the silicon-phosphorus mixture, free electrons migrate toward the positive voltage end.
- Phosphorus Electrons Configuration
- Phosphorus Electron Notation
- Phosphorus Electrons Gained Or Lost
- Phosphorus Electron Shell
- Phosphorus Electrons And Neutrons
- Phosphorus Electrons Lewis Dot Structure
Phosphorus is a chemical element with atomic number 15 which means there are 15 protons and 15 electrons in the atomic structure.
Our editors will review what you’ve submitted and determine whether to revise the article.
Join Britannica's Publishing Partner Program and our community of experts to gain a global audience for your work!Phosphorus (P), nonmetallic chemical element of the nitrogen family (Group 15 [Va] of the periodic table) that at room temperature is a colourless, semitransparent, soft, waxy solid that glows in the dark.
atomic number | 15 |
---|---|
atomic weight | 30.9738 |
melting point (white) | 44.1 °C (111.4 °F) |
boiling point (white) | 280 °C (536 °F) |
density (white) | 1.82 gram/cm3 at 20 °C (68 °F) |
oxidation states | −3, +3, +5 |
electron configuration | 1s22s22p63s23p3 |
History
Arabian alchemists of the 12th century may have isolated elemental phosphorus by accident, but the records are unclear. Phosphorus appears to have been discovered in 1669 by Hennig Brand, a German merchant whose hobby was alchemy. Brand allowed 50 buckets of urine to stand until they putrified and “bred worms.” He then boiled the urine down to a paste and heated it with sand, thereby distilling elemental phosphorus from the mixture. Brand reported his discovery in a letter to Gottfried Wilhelm Leibniz, and, thereafter, demonstrations of this element and its ability to glow in the dark, or “phosphoresce,” excited public interest. Phosphorus, however, remained a chemical curiosity until about a century later when it proved to be a component of bones. Digestion of bones with nitric or sulfuric acid formed phosphoric acid, from which phosphorus could be distilled by heating with charcoal. In the late 1800s James Burgess Readman of Edinburgh developed an electric furnace method for producing the element from phosphate rock, which is essentially the method employed today.
Occurrence and distribution
Phosphorus is a very widely distributed element—12th most abundant in crustEarth’s , to which it contributes about 0.10 weight percent. Its cosmic abundance is about one atom per 100 atoms of silicon, the standard. Its high chemical reactivity assures that it does not occur in the free state (except in a few meteorites). Phosphorus always occurs as the phosphateion. The principal combined forms in nature are the phosphate salts. About 550 different minerals have been found to contain phosphorus, but, of these, the principal source of phosphorus is the apatite series in which calcium ions exist along with phosphate ions and variable amounts of fluoride, chloride, or hydroxide ions, according to the formula [Ca10(PO4)6(F, Cl, or OH)2]. Other important phosphorus-bearing minerals are wavellite and vivianite. Commonly, such metal atoms as magnesium, manganese, strontium, and lead substitute for calcium in the mineral, and silicate, sulfate, vanadate, and similar anions substitute for phosphate ions. Very large sedimentary deposits of fluoroapatite are found in many parts of Earth. The phosphate of bone and tooth enamel is hydroxyapatite. (The principle of lessening tooth decay by fluoridation depends upon the conversion of hydroxyapatite to the harder, more decay-resistant, fluoroapatite.)
The chief commercial source is phosphorite, or phosphate rock, an impure massive form of carbonate-bearing apatite. Estimates of the total phosphate rock in Earth’s crust average about 65,000,000,000 tons, of which Morocco and Western Sahara contain about 80 percent. This estimate includes only ore that is sufficiently rich in phosphate for conversion to useful products by present methods. Vast quantities of material lower in phosphorus content also exist.
The only naturally occurring isotope of phosphorus is that of mass 31. The other isotopes from mass 24 to mass 46 have been synthesized by appropriate nuclear reactions. All of these are radioactive with relatively short half-lives. The isotope of mass 32 has a half-life of 14.268 days and has proven extremely useful in tracer studies involving the absorption and movement of phosphorus in living organisms.
Commercial production and uses
The principal technique for converting phosphate rock to usable materials involves acidulation of the crushed rock—with either sulfuric or phosphoric acids—to form crude calciumhydrogen phosphates that, being water-soluble, are valuable additions to fertilizer. Most of the output is burned to phosphoric anhydride and subsequently treated with water to form phosphoric acid, H3PO4. About 95 percent of the phosphate rock mined in the United States is used to make fertilizer or food supplements for animals. Concerns have arisen about phosphorus use, however. Most of the phosphorus is wasted on its journey from mining to being eaten by humans, and the wasted phosphorus ends up in waterways where it can cause algal blooms. Another concern is that increased phosphorus usage will deplete the nonrenewable supply of phosphate rock.
Only about 5 percent of the phosphorus consumed per year in the United States is used in the elemental form. Pyrotechnic applications of the element include tracers, incendiaries, fireworks, and matches. Some is used as an alloying agent, some is used to kill rodents, and the rest is employed in chemical synthesis. A large amount is converted to sulfides used in matches and in the manufacture of insecticides and oil additives. Most of the remainder is converted to halides or oxides for subsequent use in synthesizing organic phosphorus compounds.
- key people
- related topics
The Chemistry of Nitrogen and Phosphorous
The Chemistry of Nitrogen | The Synthesis of Ammonia | The Synthesis of Nitric Acid |
Intermediate Oxidation Numbers | Negative Oxidation Numbers of Nitrogen Besides -3 | Positive Oxidation Numbers for Nitrogen: The Nitrogen Halides |
Positive Oxidation Numbers for Nitrogen: The Nitrogen Oxides | The Chemistry of Phosphorus | The Effect of the Differences in the Singe and Triple Bond Strengths |
The Effect of Differences in the Strengths of P=X and N=X Double Bonds | The Effect of Differences in the Electronegativities of Phosphorus and Nitrogen | The Effect of Differences in the Abilities of Phosphorus and Nitrogen to Expand Their Valence Shell |
The chemistry of nitrogen is dominated by the ease with which nitrogen atoms form double and triple bonds. A neutral nitrogen atom contains five valence electrons: 2s2 2p3. A nitrogen atom can therefore achieve an octet of valence electrons by sharing three pairs of electrons with another nitrogen atom.
Because the covalent radius of a nitrogen atom is relatively small (only 0.070 nm), nitrogen atoms come close enough together to form very strong bonds. The bond-dissociation enthalpy for the nitrogen-nitrogen triple bond is 946 kJ/mol, almost twice as large as that for an O=O double bond.
The strength of the nitrogen-nitrogen triple bond makes the N2 molecule very unreactive. N2 is so inert that lithium is one of the few elements with which it reacts at room temperature.
In spite of the fact that the N2 molecule is unreactive, compounds containing nitrogen exist for virtually every element in the periodic table except those in Group VIIIA (He, Ne, Ar, and so on). This can be explained in two ways. First, N2 becomes significantly more reactive as the temperature increases. At high temperatures, nitrogen reacts with hydrogen to form ammonia and with oxygen to form nitrogen oxide.
N2(g) | + | 3 H2(g) | 2 NH3(g) |
N2(g) | + | O2(g) | 2 NO(g) |
Second, a number of catalysts found in nature overcome the inertness of N2 at low temperature.
It is difficult to imagine a living system that does not contain nitrogen, which is an essential component of the proteins, nucleic acids, vitamins, and hormones that make life possible. Animals pick up the nitrogen they need from the plants or other animals in their diet. Plants have to pick up their nitrogen from the soil, or absorb it as N2 from the atmosphere. The concentration of nitrogen in the soil is fairly small, so the process by which plants reduce N2 to NH3 or 'fix' N2 is extremely important.
Although 200 million tons of NH3 are produced by nitrogen fixation each year, plants, by themselves, cannot reduce N2 to NH3. This reaction is carried out by blue-green algae and bacteria that are associated with certain plants. The best-understood example of nitrogen fixation involves the rhizobium bacteria found in the root nodules of legumes such as clover, peas and beans. These bacteria contain a nitrogenase enzyme, which is capable of the remarkable feat of reducing N2 from the atmosphere to NH3 at room temperature.
Ammonia is made on an industrial scale by a process first developed between 1909 and 1913 by Fritz Haber. In the Haber process, a mixture of N2 and H2 gas at 200 to 300 atm and 400 to 600oC is passed over a catalyst of finely divided iron metal.
Almost 20 million tons of NH3 are produced in the United States each year by this process. About 80% of it, worth more than $2 billion, is used to make fertilizers for plants that can't fix nitrogen from the atmosphere. On the basis of weight, ammonia is the second most important industrial chemical in the United States. (Only sulfuric acid is produced in larger quantities.)
Two-thirds of the ammonia used for fertilizers is converted into solids such as ammonium nitrate, NH4NO3; ammonium phosphate, (NH4)3PO4; ammonium sulfate, (NH4)2SO4; and urea, H2NCONH2. The other third is applied directly to the soil as anhydrous (literally, 'without water') ammonia. Ammonia is a gas at room temperature. It can be handled as a liquid when dissolved in water to form an aqueous solution. Alternatively, it can be cooled to temperatures below -33oC, in which case the gas condenses to form the anhydrous liquid, NH3(l).
The NH3 produced by the Haber process that is not used as fertilizer is burned in oxygen to generate nitrogen oxide.
4 NH3(g) | + | 5 O2(g) | 4 NO(g) | + | 6 H2O(g) |
Nitrogen oxideor nitric oxide, as it was once known is a colorless gas that reacts rapidly with oxygen to produce nitrogen dioxide, a dark brown gas.
Nitrogen dioxide dissolves in water to give nitric acid and NO, which can be captured and recycled.
3 NO2(g) | + | H2O(l) | 2 HNO3(aq) | + | NO(g) |
Thus, by a three-step process developed by Friedrich Ostwald in 1908, ammonia can be converted into nitric acid.
4 NH3(g) | + | 5 O2(g) | 4 NO(g) | + | 6 H2O(g) |
2 NO(g) | + | O2(g) | 2 NO2(g) | ||
3 NO2(g) | + | H2O(l) | 2 HNO3(aq) | + | NO(g) |
The Haber process for the synthesis of ammonia combined with the Ostwald process for the conversion of ammonia into nitric acid revolutionized the explosives industry. Nitrates have been important explosives ever since Friar Roger Bacon mixed sulfur, saltpeter, and powdered carbon to make gunpowder in 1245.
16 KNO3(s) | + | S8(s) | + | 24 C(s) | 8 K2S(s) | + | 24 CO2(g) | + | 8 N2(g) | Ho = -571.9 kJ/mol N2 |
Before the Ostwald process was developed the only source of nitrates for use in explosives was naturally occurring minerals such as saltpeter, which is a mixture of NaNO3 and KNO3. Once a dependable supply of nitric acid became available from the Ostwald process, a number of nitrates could be made for use as explosives. Combining NH3 from the Haber process with HNO3 from the Ostwald process, for example, gives ammonium nitrate, which is both an excellent fertilizer and a cheap, dependable explosive commonly used in blasting powder.
The destructive power of ammonia nitrate is apparent in photographs of the Alfred P. Murrah Federal Building in Oklahoma City, which was destroyed with a bomb made from ammonium nitrate on April 19, 1995.
Nitric acid (HNO3) and ammonia (NH3) represent the maximum (+5) and minimum (-3) oxidation numbers for nitrogen. Nitrogen also forms compounds with every oxidation number between these extremes (see table below).
Common Oxidation Numbers for Nitrogen
Oxidation Number | Examples |
-3 | NH3, NH4+, NH2-, Mg3N2 |
-2 | N2H4 |
-1 | NH2OH |
-1/3 | NaN3, HN3 |
0 | N2 |
+1 | N2O |
+2 | NO, N2O2 |
+3 | HNO2, NO2-, N2O3, NO+ |
+4 | NO2, N2O4 |
+5 | HNO3, NO3-, N2O5 |
At about the time that Haber developed the process for making ammonia and Ostwald worked out the process for converting ammonia into nitric acid, Raschig developed a process that used the hypochlorite (OCl-) ion to oxidize ammonia to produce hydrazine, N2H4.
This reaction can be understood by noting that the OCl- ion is a two-electron oxidizing agent. The loss of a pair of electrons and a pair of H+ ions by neighboring NH3 molecules would produce a pair of highly reactive NH2 molecules, which would combine to form a hydrazine molecule as shown in the figure below.
Hydrazine is a colorless liquid with a faint odor of ammonia that can be collected when this solution is heated until N2H4 distills out of the reaction flask. Many of the physical properties of hydrazine are similar to those of water.
H2O | N2H4 | |
Density | 1.000 g/cm3 | 1.008 g/cm3 |
Melting Point | 0.00oC | 1.54oC |
Boiling Point | 100oC | 113.8oC |
There is a significant difference between the chemical properties of these compounds, however. Hydrazine burns when ignited in air to give nitrogen gas, water vapor, and large amounts of energy.
N2H4(l) | + | O2(g) | N2(g) | + | 2 H2O(g) | Ho = -534.3 kJ/mol N2H4 |
The principal use of hydrazine is as a rocket fuel. It is second only to liquid hydrogen in terms of the number of kilograms of thrust produced per kilogram of fuel burned. Hydrazine has several advantages over liquid H2, however. It can be stored at room temperature, whereas liquid hydrogen must be stored at temperatures below -253oC. Hydrazine is also more dense than liquid H2 and therefore requires less storage space.
Pure hydrazine is seldom used as a rocket fuel because it freezes at the temperatures encountered in the upper atmosphere. Hydrazine is mixed with N,N-dimethylhydrazine, (CH3)2NNH2, to form a solution that remains a liquid at low temperatures. Mixtures of hydrazine and N,N-dimethylhydrazine were used to fuel the Titan II rockets that carried the Project Gemini spacecraft, and the reaction between hydrazine derivatives and N2O4 is still used to fuel the small rocket engines that enable the space shuttle to maneuver in space.
The product of the combustion of hydrazine is unusual. When carbon compounds burn, the carbon is oxidized to CO or CO2. When sulfur compounds burn, SO2 is produced. When hydrazine is burned, the product of the reaction is N2 because of the unusually strong nitrogen-nitrogen triple bond in the N2 molecule.
Phosphorus Electrons Configuration
Hydrazine reacts with nitrous acid (HNO2) to form hydrogen azide, HN3, in which the nitrogen atom formally has an oxidation state of -1/3.
N2H4(aq) | + | HNO2(aq) | HN3(aq) | + | 2 H2O(l) |
Pure hydrogen azide is an extremely dangerous substance. Even dilute solutions should be handled with care because of the risk of explosions. Hydrogen azide is best described as a resonance hybrid of the Lewis structures shown in the figure below. The corresponding azide ion, N3-, is a linear molecule, which is a resonance hybrid of three Lewis structures.
Fluorine, oxygen, and chlorine are the only elements more electronegative than nitrogen. As a result, positive oxidation numbers of nitrogen are found in compounds that contain one or more of these elements.
In theory, N2 could react with F2 to form a compound with the formula NF3. In practice, N2 is too inert to undergo this reaction at room temperature. NF3 is made by reacting ammonia with F2 in the presence of a copper metal catalyst.
Cu | |||||
NH3(g) | + | 3 F2(g) | NF3(g) | + | 3 HF(g) |
The HF produced in this reaction combines with ammonia to form ammonium fluoride. The overall stoichiometry for the reaction is therefore written as follows.
The Lewis structure of NF3 is analogous to the Lewis structure of NH3, and the two molecules have similar shapes.
Ammonia reacts with chlorine to form NCl3, which seems at first glance to be closely related to NF3. But there is a significant difference between these compounds. NF3 is essentially inert at room temperature, whereas NCl3 is a shock-sensitive, highly explosive liquid that decomposes to form N2 and Cl2.
2 NCl3(l) | N2(g) | + | 3 Cl2(g) |
Ammonia reacts with iodine to form a solid that is a complex between NI3 and NH3. This material is the subject of a popular, but dangerous, demonstration in which freshly prepared samples of NI3 in ammonia are poured onto filter paper, which is allowed to dry on a ring stand. After the ammonia evaporates, the NH3/NI3 crystals are touched with a feather attached to a meter stick, resulting in detonation of this shock-sensitive solid, which decomposes to form a mixture of N2 and I2.
Lewis structures for seven oxides of nitrogen with oxidation numbers ranging from +1 to +5 are given in the table below.
These compounds all have two things in common: they contain N=O double bonds and they are less stable than their elements in the gas phase, as shown by the enthalpy of formation data in the table below.
Enthalpy of Formation Data for the Oxides of Nitrogen
Compound | Hof (kJ/mol) |
N2O(g) | 82.05 |
NO(g) | 90.25 |
NO2(g) | 33.18 |
N2O3(g) | 83.72 |
N2O4(g) | 9.16 |
N2O5(g) | 11.35 |
Dinitrogen oxide, N2O, which is also known as nitrous oxide, can be prepared by carefully decomposing ammonium nitrate.
Nitrous oxide is a sweet-smelling, colorless gas best known to nonchemists as 'laughing gas.' As early as 1800, Humphry Davy noted that N2O, inhaled in relatively small amounts, produced a state of apparent intoxication often accompanied by either convulsive laughter or crying. When taken in larger doses, nitrous oxide provides fast and efficient relief from pain. N2O was therefore used as the first anesthetic. Because large doses are needed to produce anesthesia, and continued exposure to the gas can be fatal, N2O is used today only for relatively short operations.
Nitrous oxide has several other interesting properties. First, it is highly soluble in cream; for that reason, it is used as the propellant in whipped cream dispensers. Second, although it does not burn by itself, it is better than air at supporting the combustion of other objects. This can be explained by noting that N2O can decompose to form an atmosphere that is one-third O2 by volume, whereas normal air is only 21% oxygen by volume.
2 N2O(g) | 2 N2(g) | + | O2(g) |
For many years, the endings -ous and -ic were used to distinguish between the lowest and highest of a pair of oxidation numbers. N2O is nitrous oxide because the oxidation number of the nitrogen is +1. NO is nitric oxide because the oxidation number of the nitrogen is +2.
Enormous quantities of nitrogen oxide, or nitric oxide, are generated each year by the reaction between the N2 and O2 in the atmosphere, catalyzed by a stroke of lightning passing through the atmosphere or by the hot walls of an internal combustion engine.
One of the reasons for lowering the compression ratio of automobile engines in recent years is to decrease the temperature of the combustion reaction, thereby decreasing the amount of NO emitted into the atmosphere.
NO can be prepared in the laboratory by reacting copper metal with dilute nitric acid.
3 Cu(s) | + | 8 HNO3(aq) | 3 Cu(NO3)2(aq) | + | 2 NO(g) | + | 4 H2O(l) |
The NO molecule contains an odd number of valence electrons. As a result, it is impossible to write a Lewis structure for this molecule in which all of the electrons are paired (see table of oxides of nitrogen). When NO gas is cooled, pairs of NO molecules combine in a reversible reaction to form a dimer (from the Greek, 'two parts'), with the formula N2O2, in which all of the valence electrons are paired, as shown in the table of oxides of nitrogen.
NO reacts rapidly with O2 to form nitrogen dioxide (once known as nitrogen peroxide), which is a dark brown gas at room temperature.
NO2 can be prepared in the laboratory by heating certain metal nitrates until they decompose.
2 Pb(NO3)2(s) | 2 PbO(s) | + | 4 NO2(g) | + | O2(g) |
It can also be made by reacting copper metal with concentrated nitric acid,
NO2 also has an odd number of electrons and therefore contains at least one unpaired electron in its Lewis structures. NO2 dimerizes at low temperatures to form N2O4 molecules, in which all the electrons are paired, as shown in the table of oxides of nitrogen.
Mixtures of NO and NO2 combine when cooled to form dinitrogen trioxide, N2O3, which is a blue liquid. The formation of a blue liquid when either NO or NO2 is cooled therefore implies the presence of at least a small portion of the other oxide because N2O2 and N2O4 are both colorless.
By carefully removing water from concentrated nitric acid at low temperatures with a dehydrating agent we can form dinitrogen pentoxide.
4 HNO3(aq) | + | P4O10(s) | 2 N2O5(s) | + | 4 HPO3(s) |
N2O5 is a colorless solid that decomposes in light or on warming to room temperature. As might be expected, N2O5 dissolves in water to form nitric acid.
Phosphorus is the first element whose discovery can be traced to a single individual. In 1669, while searching for a way to convert silver into gold, Hennig Brand obtained a white, waxy solid that glowed in the dark and burst spontaneously into flame when exposed to air. Brand made this substance by evaporating the water from urine and allowing the black residue to putrefy for several months. He then mixed this residue with sand, heated this mixture in the presence of a minimum of air, and collected under water the volatile products that distilled out of the reaction flask.
Phosphorus forms a number of compounds that are direct analogs of nitrogen-containing compounds. However, the fact that elemental nitrogen is virtually inert at room temperature, whereas elemental phosphorus can burst spontaneously into flame when exposed to air, shows that there are differences between these elements as well. Phosphorus often forms compounds with the same oxidation numbers as the analogous nitrogen compounds, but with different formulas, as shown in the table below.
Nitrogen and Phosphorus Compounds with the Same Oxidation Numbers but Different Formulas
Oxidation Number | Nitrogen Compound | Phosphorus Compound |
0 | N2 | P4 |
+3 | HNO2 (nitrous acid) | H3PO3 (phosphorous acid) |
+3 | N2O3 | P4O6 |
+5 | HNO3 (nitric acid) | H3PO4 (phosphoric acid) |
+5 | NaNO3 (sodium nitrate) | Na3PO4 (sodium phosphate) |
+5 | N2O5 | P4O10 |
The same factors that explain the differences between sulfur and oxygen can be used to explain the differences between phosphorus and nitrogen.
1. Nitrogen-nitrogen triple bonds are much stronger than phosphorus-phosphorus triple bonds.
2. P-P single bonds are stronger than N-N single bonds.
3. Phosphorus (EN = 2.19) is much less electronegative than nitrogen (EN = 3.04).
4. Phosphorus can expand its valence shell to hold more than eight electrons, but nitrogen cannot.
Phosphorus Electron Notation
The ratio of the radii of phosphorus and nitrogen atoms is the same as the ratio of the radii of sulfur and oxygen atoms, within experimental error.
As a result, phosphorus-phosphorus triple bonds are much weaker than Nitrogen-nitrogen triple bonds, for the same reason that S=S double bonds are weaker than O=O double bonds phosphorus atoms are too big to come close enough together to form strong bonds.
Each atom in an N2 molecule completes its octet of valence electrons by sharing three pairs of electrons with a single neighboring atom. Because phosphorus does not form strong multiple bonds with itself, elemental phosphorus consists of tetrahedral P4 molecules in which each atom forms single bonds with three neighboring atoms, as shown in the figure below.
Phosphorus is a white solid with a waxy appearance, which melts at 44.1oC and boils at 287oC. It is made by reducing calcium phosphate with carbon in the presence of silica (sand) at very high temperatures.
2 Ca3(PO4)2(s) | + | 6 SiO2(s) | + | 10 C(s) | 6 CaSiO3(s) | + | P4(s) | + | 10 CO(g) |
White phosphorus is stored under water because the element spontaneously bursts into flame in the presence of oxygen at temperatures only slightly above room temperature. Although phosphorus is insoluble in water, it is very soluble in carbon disulfide. Solutions of P4 in CS2 are reasonably stable. As soon as the CS2 evaporates, however, the phosphorus bursts into flame.
The P-P-P bond angle in a tetrahedral P4 molecule is only 60o. This very small angle produces a considerable amount of strain in the P4 molecule, which can be relieved by breaking one of the P-P bonds. Phosphorus therefore forms other allotropes by opening up the P4 tetrahedron. When white phosphorus is heated to 300oC, one bond inside each P4 tetrahedron is broken, and the P4 molecules link together to form a polymer (from the Greek pol, 'many,' and meros, 'parts') with the structure shown in the figure below. This allotrope of phosphorus is dark red, and its presence in small traces often gives white phosphorus a light yellow color. Red phosphorus is more dense (2.16 g/cm3) than white phosphorus (1.82 g/cm3) and is much less reactive at normal temperatures.
The size of a phosphorus atom also interferes with its ability to form double bonds to other elements, such as oxygen, nitrogen, and sulfur. As a result, phosphorus tends to form compounds that contain two P-O single bonds where nitrogen would form an N=O double bond. Nitrogen forms the nitrate, NO3-, ion, for example, in which it has an oxidation number of +5. When phosphorus forms an ion with the same oxidation number, it is the phosphate, PO43-, ion, as shown in the figure below.
Similarly, nitrogen forms nitric acid, HNO3, which contains an N=O double bond, whereas phosphorus forms phosphoric acid, H3PO4, which contains P-O single bonds, as shown in the figure below.
The difference between the electronegativities of phosphorus and nitrogen (EN = 0.85) is the same as the difference between the electronegativities of sulfur and oxygen (EN = 0.86), within experimental error. Because it is less electronegative, phosphorus is more likely than nitrogen to exhibit positive oxidation numbers. The most important oxidation numbers for phosphorus are -3, +3, and +5 (see table below).
Common Oxidation Numbers of Phosphorus
Oxidation Number | Examples |
-3 | Ca3P2, PH3 |
+3 | PF3, P4O10, H3PO3 |
+5 | PF5, P4O10, H3PO4, PO43- |
Because it is more electronegative than most metals, phosphorus reacts with metals at elevated temperatures to form phosphides, in which it has an oxidation number of -3.
Phosphorus Electrons Gained Or Lost
These metal phosphides react with water to produce a poisonous, highly reactive, colorless gas known as phosphine (PH3), which has the foulest odor the authors have encountered.
Ca3P2(s) | + | 6 H2O(l) | 2 PH3(g) | + | 3 Ca2+(aq) | + | 6 OH-(aq) |
Samples of PH3, the phosphorus analog of ammonia, are often contaminated by traces of P2H4, the phosphorus analog of hydrazine. As if the toxicity and odor of PH3 were not enough, mixtures of PH3 and P2H4 burst spontaneously into flame in the presence of oxygen.
Compounds (such as Ca3P2 and PH3) in which phosphorus has a negative oxidation number are far outnumbered by compounds in which the oxidation number of phosphorus is positive. Phosphorus burns in O2 to produce P4O10 in a reaction that gives off extraordinary amounts of energy in the form of heat and light.
When phosphorus burns in the presence of a limited amount of O2, P4O6 is produced.
P4(s) | + | 3 O2(g) | P4O6(s) | Ho = -1640 kJ/mol P4 |
P4O6 consists of a tetrahedron in which an oxygen atom has been inserted into each P-P bond in the P4 molecule (see figure below). P4O10 has an analogous structure, with an additional oxygen atom bound to each of the four phosphorus atoms.
P4O6 and P4O10 react with water to form phosphorous acid, H3PO3, and phosphoric acid, H3PO4, respectively.
Phosphorus Electron Shell
P4O6(s) | + | 6 H2O(l) | 4 H3PO3(aq) |
P4O10(s) | + | 6 H2O(l) | 4 H3PO4(aq) |
Phosphorus Electrons And Neutrons
P4O10 has such a high affinity for water that it is commonly used as a dehydrating agent. Phosphorous acid, H3PO3, and phosphoric acid, H3PO4, are examples of a large class of oxyacids of phosphorus. Lewis structures for some of these oxyacids and their related oxyanions are given in the table below.
The reaction between ammonia and fluorine stops at NF3 because nitrogen uses the 2s, 2px, 2py and 2pz orbitals to hold valence electrons. Nitrogen atoms can therefore hold a maximum of eight valence electrons. Phosphorus, however, has empty 3d atomic orbitals that can be used to expand the valence shell to hold 10 or more electrons. Thus, phosphorus can react with fluorine to form both PF3 and PF5. Phosphorus can even form the PF6- ion, in which there are 12 valence electrons on the central atom, as shown in the figure below.
Phosphorus Electrons Lewis Dot Structure
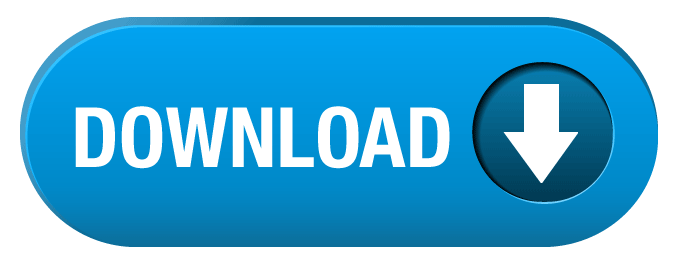